In this lesson, we’ll discuss how to identify the most acidic proton in a molecule. This topic is frequently addressed in the acid-based chapters of textbooks and is a common exam question. As such, you can expect it to appear on future tests, finals, and standardized exams such as the ACS or MCAT.
Understanding where the acidic protons is vital. To determine the most acidic proton in your molecule, start by identifying the protons you believe to be the most acidic. Label them as proton “A”, proton “B”, and proton “C”, etc. Then, separate these protons from your molecule to form the corresponding conjugate bases and analyze their properties.
You are viewing: Which Molecule Is Most Acidic
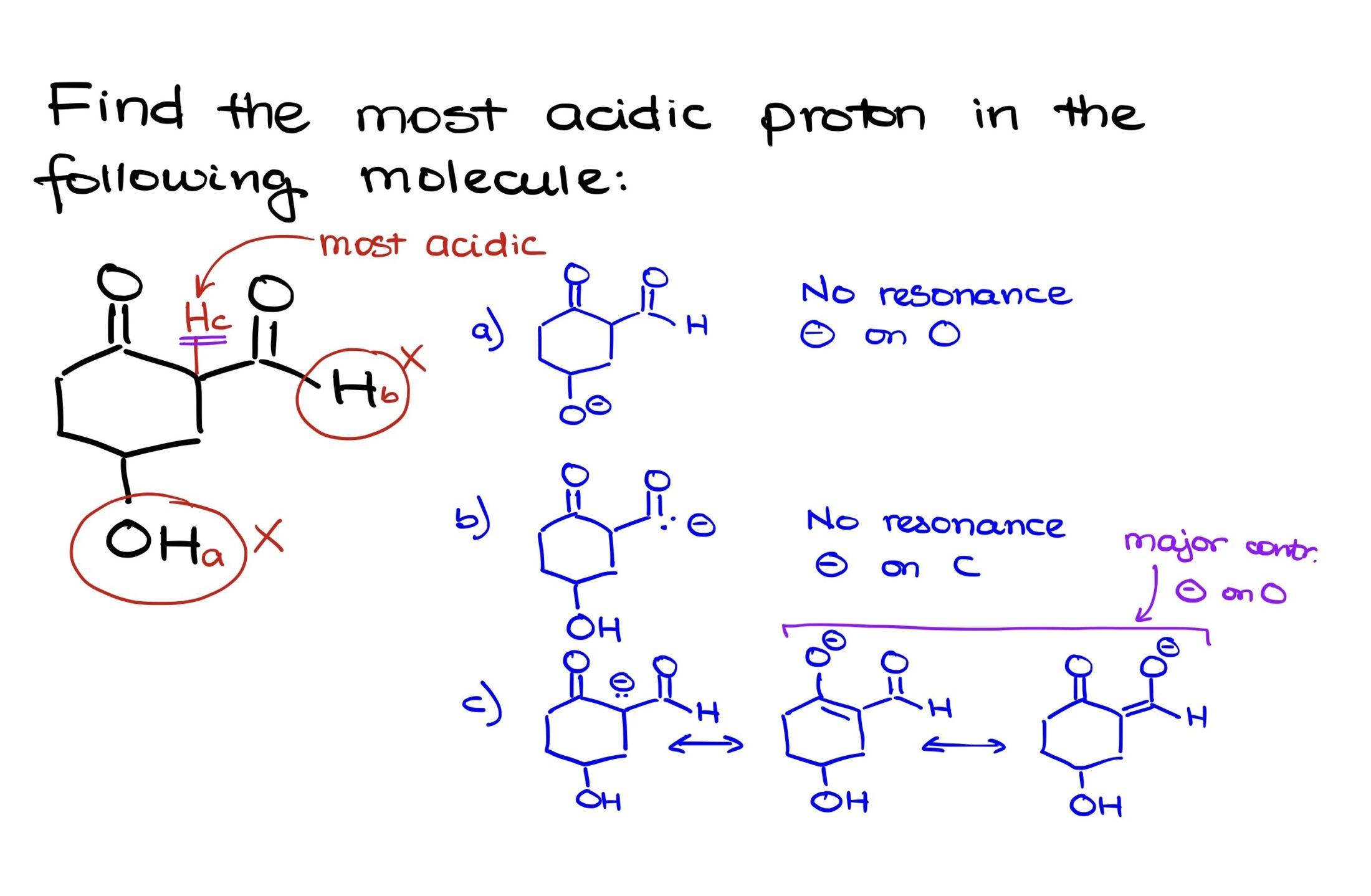
For instance, if we remove proton “A”, the resulting conjugate base features a charge on the oxygen. Notably, this charge is localized, indicating a lack of resonance stabilization. This absence of resonance is not ideal; it’s generally preferred to have resonance stabilization, especially with negatively charged species, as it contributes to stability.
Removing proton “B” results in a conjugate base where the negative charge resides on carbon, an element not renowned for its electronegativity. This makes it a poor stabilizer for the negative charge, especially given the absence of any resonance.
However, when proton “C” is removed, the resultant conjugate base displays resonance contributors that stabilize the negative charge. We can illustrate two primary resonance contributors, both of which position the negative charge on an oxygen atom. Due to the presence and significance of these contributors, the negative charge is well stabilized.
This stabilization indicates that the conjugate base formed from Proton C is the most stable among the three. Thus, Proton C, situated between the carbonyls, is our most acidic proton.
Common Functional Groups with the Corresponding pKa Values
To build on our discussion, let’s delve into the factors determining the acidity of protons: resonance, atomic size, electronegativity of atoms carrying a negative charge, inductive effects, and hybridization. However, while these factors provide a general understanding, they’re not definitive rules but more like guidelines. There are numerous instances where these principles might not strictly apply.
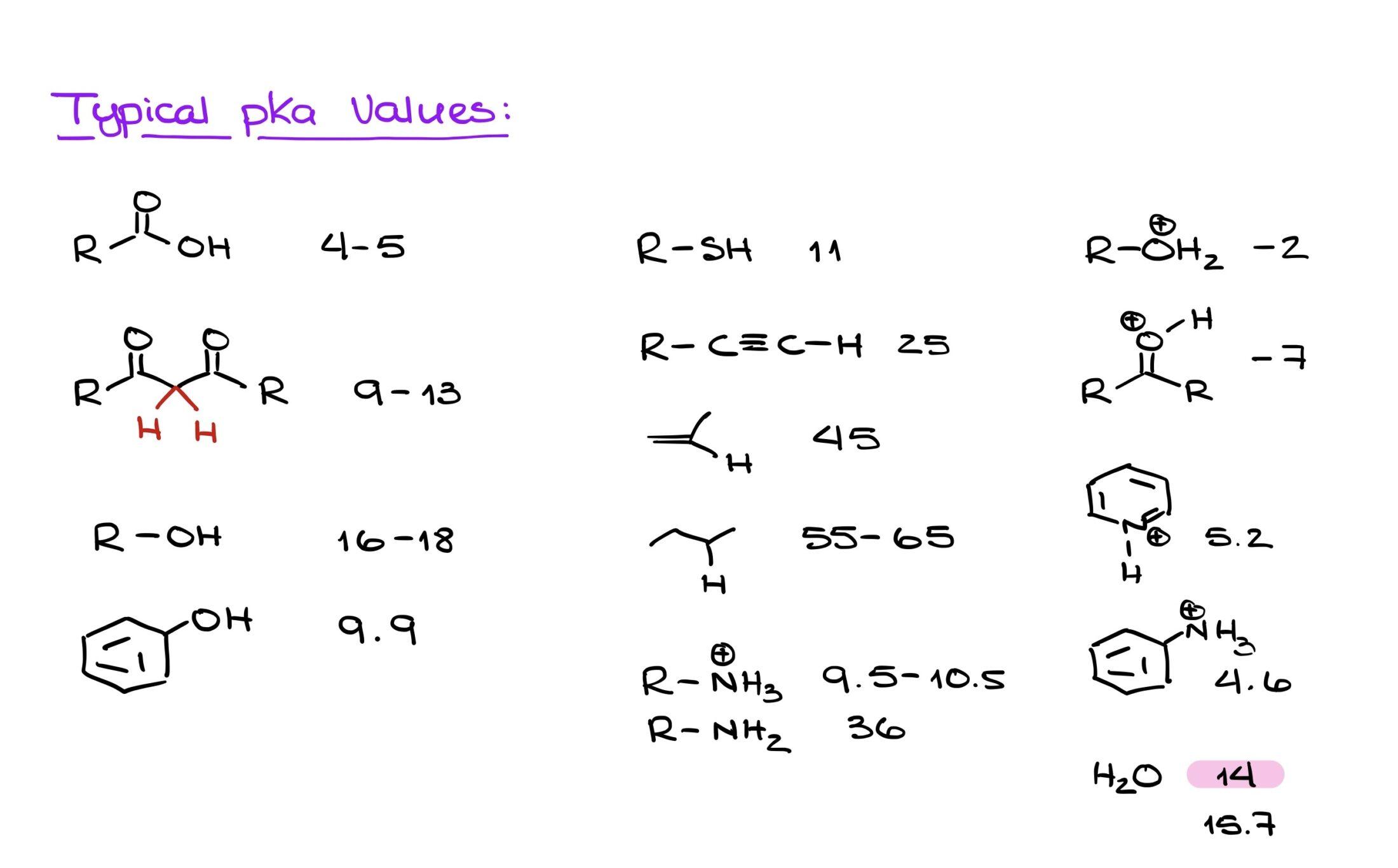
Understanding your functional groups and their pKa values is crucial. Instead of solely relying on the aforementioned factors, a more reliable method is recognizing the functional groups and being aware of the general acidity levels of these groups, i.e., which are more or less acidic.
I know it might sound daunting, but memorizing typical pKa values for common functional groups can be incredibly beneficial. While I’m not suggesting you commit the entire pKa table from your textbook or class materials to memory, knowing the values for frequent functional groups can expedite your problem-solving process. This knowledge will not only save you time but will also simplify many acid-base related questions.
To excel in organic chemistry, these are the pKa values that can serve as invaluable tools. I know it might seem like a daunting task with so many numbers to remember, but bear with me. Instead of merely attempting to memorize them, why not incorporate them into your daily routine? Create a cheat sheet or a quick reference list. Print out a few copies, place one in your organic chemistry binder, stick some around your living space, or even set it as your phone wallpaper. The key is repetition; glancing at this list daily will reinforce your memory, and you’d be surprised at how quickly these numbers become second nature.
I promise this isn’t busywork. These pKa values will recur throughout your coursework. Being familiar with the pKa values of common functional groups won’t just be a lifeline during exams and homework—it’s also a significant time-saver. Think of this not just as memorization but as an investment in streamlining your organic chemistry journey.
Examples
Example 1:
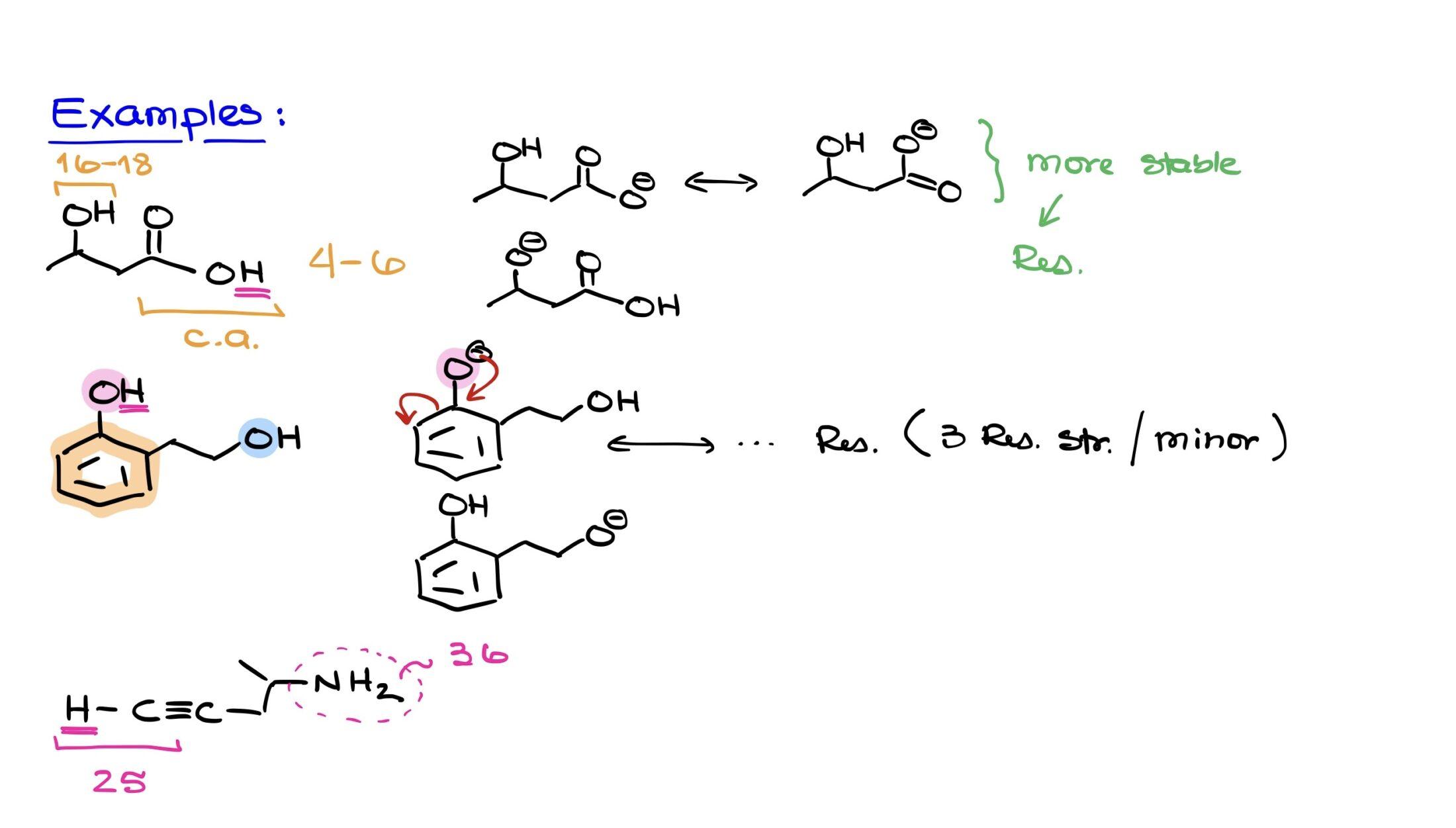
Let’s apply the factors we discussed using a practical example. Consider a molecule that comprises both an alcohol functional group and a carboxylic acid functional group. Based on our knowledge of functional groups, we recognize that the carboxylic acid is substantially more acidic than the alcohol. A typical pKa value for carboxylic acids usually ranges between 4 and 6. In contrast, alcohols generally have a pKa value between 16 and 18.
Immediately, by identifying these functional groups, we can ascertain that the carboxylic acid is markedly more acidic. Consequently, the proton on the OH group of the carboxylic acid is the most acidic proton in this molecule.
However, when faced with homework or exam questions, simply stating the known acidity or pKa values won’t suffice as reasoning. Therefore, we must delve into the foundational reasons behind these observations.
To clarify, let’s visualize the conjugate bases formed upon deprotonation. For the carboxylic acid group, its deprotonation results in a conjugate base that benefits from resonance, allowing the negative charge to be delocalized and thereby stabilized. On the other hand, deprotonating the alcohol leads to a conjugate base devoid of any resonance stabilization or any other favorable effects to stabilize the negative charge.
Read more : Which Uggs Have Removable Insoles
Given this analysis, the carboxylic acid’s conjugate base emerges as the more stable of the two, due to its resonance stabilization. This increased stability directly implies a higher acidity, further underscoring the importance of understanding resonance and other stabilizing effects.
Example 2:
In our next example, we’re presented with a molecule that has an aromatic ring accompanied by two OH groups. At first glance, it may seem we’re dealing with two alcohols. However, a closer look reveals that the “pink” OH group, positioned on top, is directly attached to the aromatic ring, thereby classifying it as a phenol. The “blue” OH group on the right side is attached to an sp3 hybridized carbon without any significant functional attachment, making it a regular alcohol.
It’s known that phenols are generally more acidic than alcohols. So, without hesitation, we can infer that the proton from the pink OH group (phenol) is more acidic than the blue one (alcohol).
To bolster this assertion with our factors, let’s compare the conjugate bases resulting from the deprotonation of both OH groups:
1. Deprotonating the pink OH group (phenol) produces a conjugate base with a negative charge on the top oxygen. This negative charge is stabilized through resonance across the aromatic ring. While we won’t draw them here, it’s worth noting that you can sketch multiple resonance structures, resulting in three additional structures. However, these are considered minor structures since the negative charge would be located on carbon atoms, disrupting the aromatic nature of the ring, thus reducing the overall stability of the molecule.
2. For the blue OH group (alcohol), deprotonation yields a conjugate base with a localized negative charge on the oxygen. This negative charge lacks resonance stabilization, meaning it’s fixed in its position. Its only redeeming quality is being located on an oxygen atom, which isn’t enough to grant it significant stability.
From this analysis, the conjugate base derived from the pink OH group (phenol) is evidently more stable. This fact underscores the reason behind the increased acidity of the pink OH group compared to the blue one.
Example 3:
In this example, we are presented with a molecule featuring a triple bond connected to a chain that includes an NH2 group. Typically, when identifying acidic protons, heteroatoms like oxygen and nitrogen are the usual suspects. Here, following that trend, one might be inclined to guess that the protons on the nitrogen would be more acidic. However, with a familiarity of the pKa values of various functional groups, we recognize that amine groups have a pKa value of approximately 36, while terminal alkynes possess a pKa value closer to 25. This considerable difference, spanning over 10 to the 10th power, underscores that the terminal alkyne is substantially more acidic in this context.
This particular scenario is interesting as it challenges some conventional expectations. Let’s dissect it further:
- Resonance: This isn’t a defining feature for either group in this instance.
- Atomic Size: This factor doesn’t differentiate the groups either since both carbon and nitrogen are in the same period, making their sizes relatively similar.
- Electronegativity: Nitrogen has a greater electronegativity than carbon. Given this, one would anticipate the nitrogen to stabilize a negative charge more effectively. However, this isn’t the case here.
The significant factor making a difference here is hybridization. The triple-bonded carbon in the terminal alkyne is sp-hybridized, which provides a unique ability to stabilize a negative charge better than the NH2 group. This hybridization factor, often overlooked, takes precedence in this example. Without the specific knowledge of the pKa values for these groups, it would be easy to mistakenly deem the NH2 group as more acidic. In reality, it’s the hydrogen of the terminal alkyne that stands out as the most acidic.
Example 4:
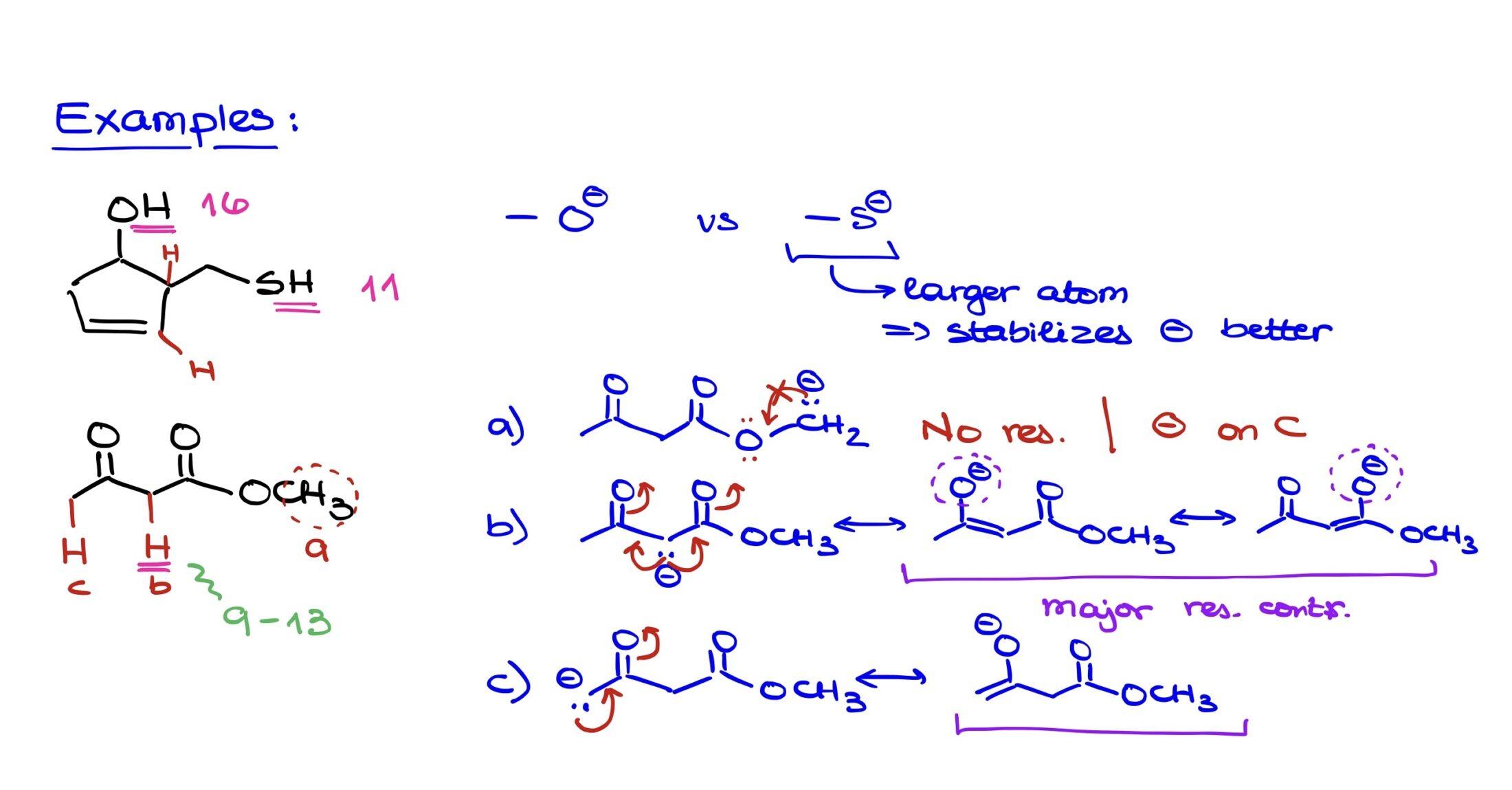
This molecule presents an interesting contrast between an alcohol (OH) and a thiol (SH) functional group. Given a basic understanding of pKa values, we can quickly discern that thiols have a typical pKa of around 11, while alcohols are slightly less acidic with a pKa of approximately 16. Hence, the thiol group is indeed more acidic.
Apart from the OH and SH, if we explore other protons in the molecule:
- The proton in a regular alkane would have a substantially higher pKa, around 50, making it much less acidic.
- The proton on an sp2 hybridized carbon, due to its hybridization and location, would have a pKa closer to 45, which again is much less acidic compared to either the alcohol or the thiol.
When comparing the acidity of the OH and SH directly and explaining it with our factors:
- Resonance: Neither group has any resonance to consider in this scenario.
- Electronegativity: Oxygen is more electronegative than sulfur, so one might initially think that the oxygen would stabilize a negative charge better. But the pKa values suggest otherwise.
- Atomic Size: Here’s where the major difference arises. Sulfur, being in the third period of the periodic table, is significantly larger than oxygen, which resides in the second period. When an atom is larger, it can spread out and stabilize a negative charge over a larger volume. This makes the negative charge on sulfur (in the conjugate base of the thiol) more stable than the negative charge on oxygen (in the conjugate base of the alcohol). This size factor, therefore, accounts for the thiol’s greater acidity.
Read more : Which Country Has The Strongest People
This example underlines the significance of atomic size in influencing acidity, particularly when considering molecules with similar functional groups but different atoms.
Example 5:
This example illustrates the importance of resonance stabilization when considering the acidity of protons in organic molecules. Here, you don’t have the usual suspects of heteroatoms bearing hydrogens, making the problem a bit more challenging.
The molecule has three potential sites where protons might be acidic:
- Proton A: Located on a carbon adjacent to an oxygen atom.
- Proton B: Situated on a carbon that’s flanked by two carbonyl groups.
- Proton C: Found on a carbon that’s next to just one carbonyl group.
Upon deprotonating these carbons:
- Conjugate Base A: This leads to a negative charge on the carbon atom. As you pointed out, there’s no potential for resonance stabilization because oxygen, being adjacent to the negatively charged carbon, already has a complete octet.
- Conjugate Base B: This is the most interesting of the three. When deprotonated, the negative charge on this carbon can be stabilized by resonance in two distinct directions, either towards the left or the right carbonyl group. This creates two additional major resonance contributors with the negative charge situated on an oxygen atom, which is more electronegative and better at stabilizing a negative charge than carbon.
- Conjugate Base C: Deprotonation results in a negative charge on a carbon adjacent to only one carbonyl group. While there’s potential for resonance stabilization, it’s limited to just one major resonance contributor. This means the negative charge is less stabilized than in the case of the conjugate base B.
Based on the resonance stabilization factors and the quality of resonance structures, it’s clear that the conjugate base of proton B is the most stabilized. Hence, proton B is the most acidic in this molecule.
This example emphasizes the importance of resonance structures in acidity determination, and it also demonstrates the concept of the ‘quality’ of resonance structures (with negative charges on more electronegative atoms being more stabilizing than those on less electronegative atoms).
Analysis of a Molecule with Two Identical Functional Groups:
In the given example, the focus is on discerning which of two identical functional groups is more acidic based on factors other than the inherent acidity of the functional group itself.
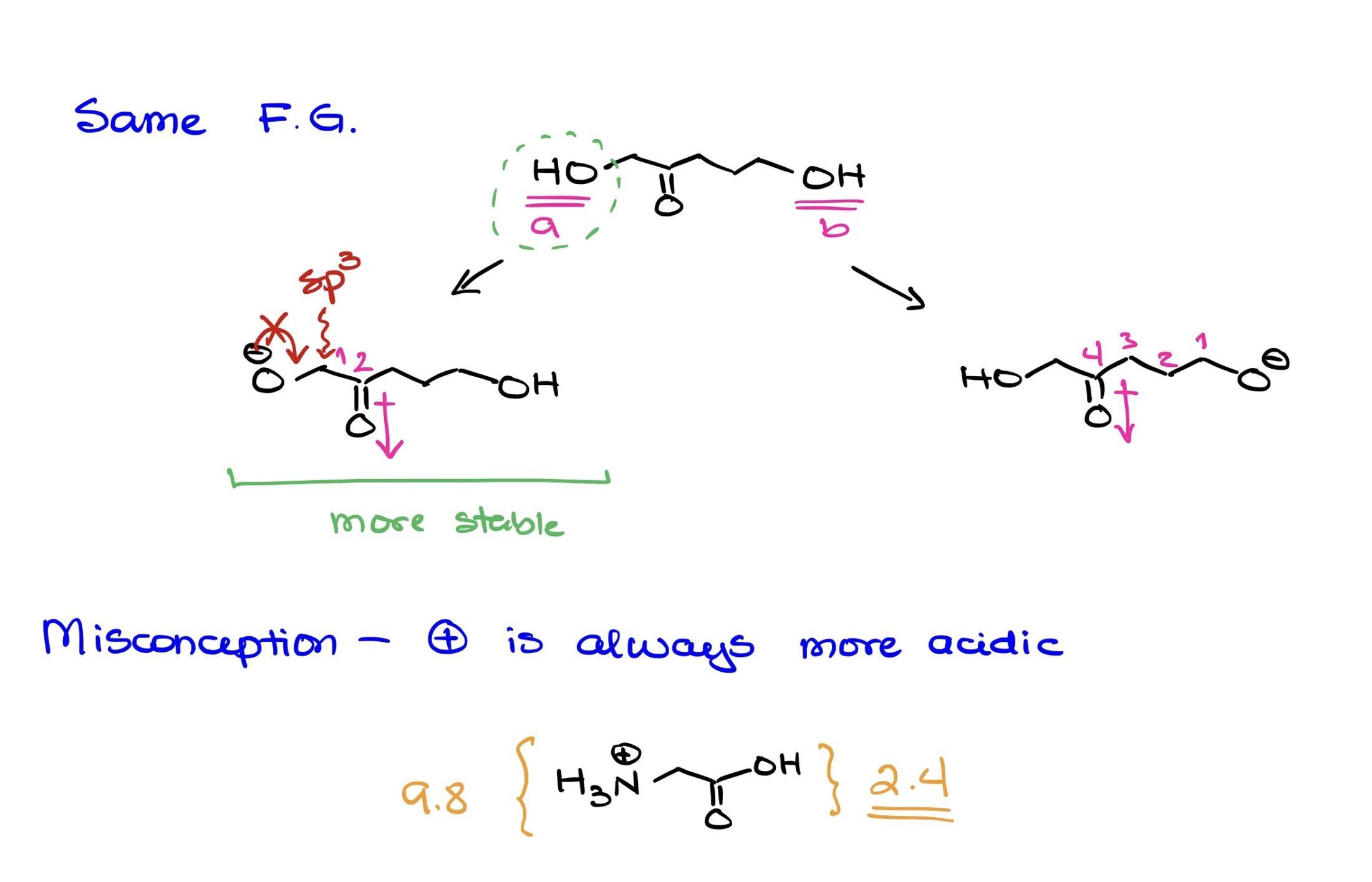
The molecule features two alcohols (OH groups), designated as Proton A and Proton B, on either side.
When considering the acidity of protons, we typically assess:
- Resonance stabilization of the resultant conjugate base.
- Electronegativity and atomic size of the atom bearing the negative charge.
- Inductive effects stemming from nearby electron-withdrawing or electron-donating groups.
For this molecule:
- Resonance: Neither deprotonated form, O-, benefits from resonance stabilization. The adjacent carbons to the oxygens are sp3-hybridized, and hence, pi-bonding to facilitate resonance isn’t possible.
- Electronegativity & Atomic Size: Both negative charges, if the protons are removed, will be on an oxygen atom. So, from an electronegativity and atomic size perspective, both are equal. Oxygen is good at stabilizing negative charges due to its high electronegativity.
- Inductive Effects: Here’s where the significant difference lies. Proton A’s O- is in closer proximity to a carbonyl group (C=O). The carbonyl group is electron-withdrawing by inductive effects due to the polarity of the C=O bond. The closeness of this carbonyl to the O- for Proton A means it can help stabilize the negative charge more effectively than in the case of Proton B, where the carbonyl is further away. Inductive effects indeed decrease in strength over distance, often notably diminishing beyond 3-5 bonds. Given that the carbonyl is closer to the O- of Proton A than it is to Proton B, the inductive stabilization is stronger in the former case.
Therefore, based on the stronger inductive stabilization from the nearby carbonyl group, Proton A’s conjugate base is more stable, making Proton A the more acidic proton in the molecule.
This analysis highlights the importance of looking at the broader molecular context when determining the acidity of protons, especially when dealing with identical functional groups. Even in cases where the inherent acidity of the functional group might be the same, the surrounding environment can significantly influence which proton is more acidic.
The Myth of Positively Charged Acidity in Organic Chemistry
Organic chemistry is a realm of intricacies, and it’s often tempting to lean on simplified rules to navigate its complexities. However, such shortcuts can sometimes lead us astray, particularly when it comes to acidity. One common misconception is that a positively charged functional group is universally more acidic than a neutral counterpart.
Debunking the Misconception
To demonstrate the fallacy of this widespread belief, let’s consider glycine, a basic amino acid. When it’s in its fully protonated state, glycine features both a carboxylic acid group and a protonated amine group. Generally, carboxylic acids have a pKa value ranging between 4 and 5. However, in glycine, due to the neighboring protonated nitrogen, the pKa of its carboxylic acid group sharply drops to 2.4.
Key Takeaways
If there’s anything to be gleaned from the above, it’s that organic chemistry doesn’t always conform to our assumptions. Here are a few points to remember:
- Question Over-simplified Rules: While they might seem helpful, shortcuts can obscure the bigger picture.
- Functional Groups Are Priority: Before diving into other factors, familiarize yourself with the inherent acidity of different functional groups.
- Assessing Acidity: When faced with identical functional groups, use additional factors like resonance and inductive effects to determine acidity levels.
- Memory Aid: While understanding principles is crucial, memorizing pKa values for common functional groups can indeed be beneficial. It provides a handy reference when you’re in a fix.
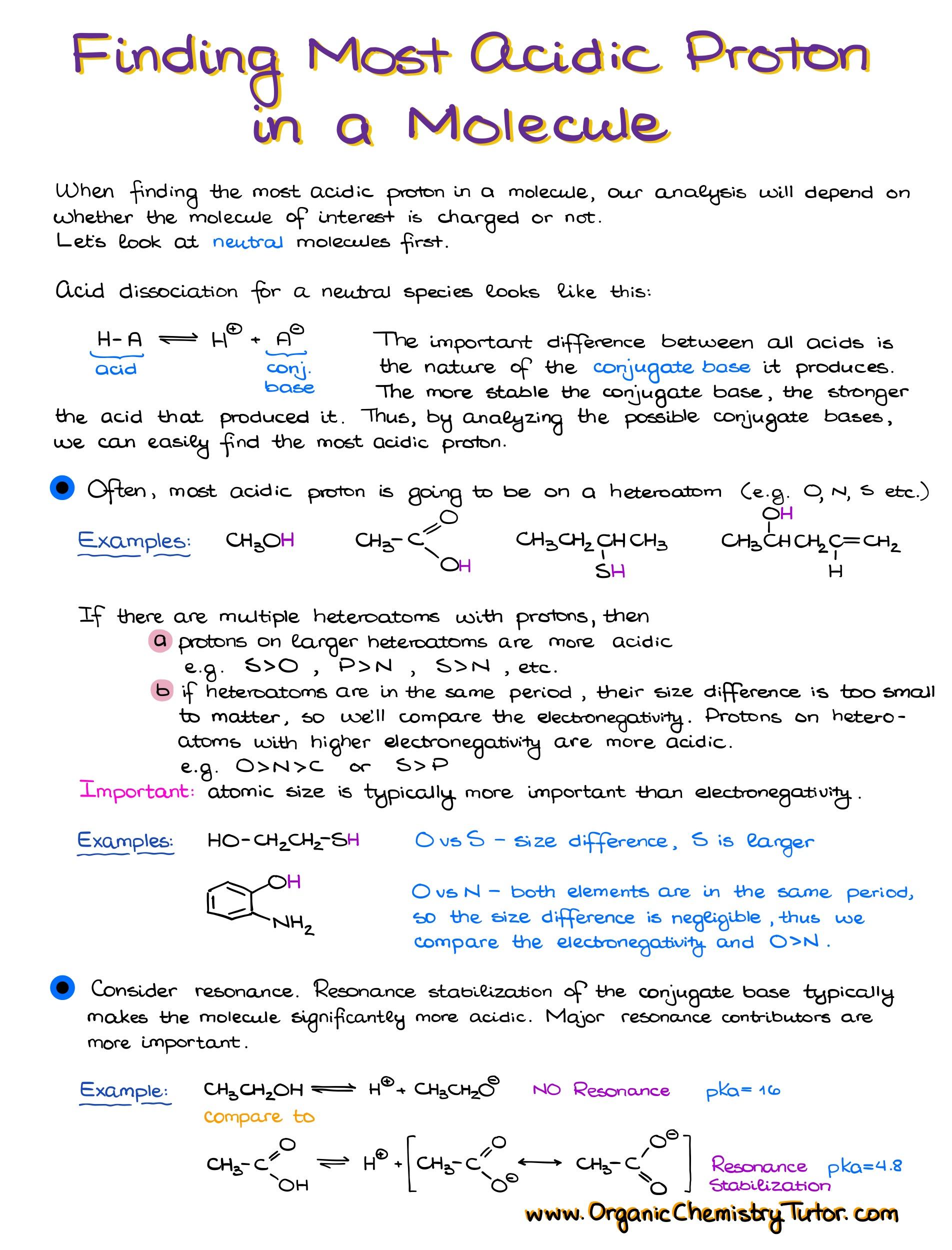
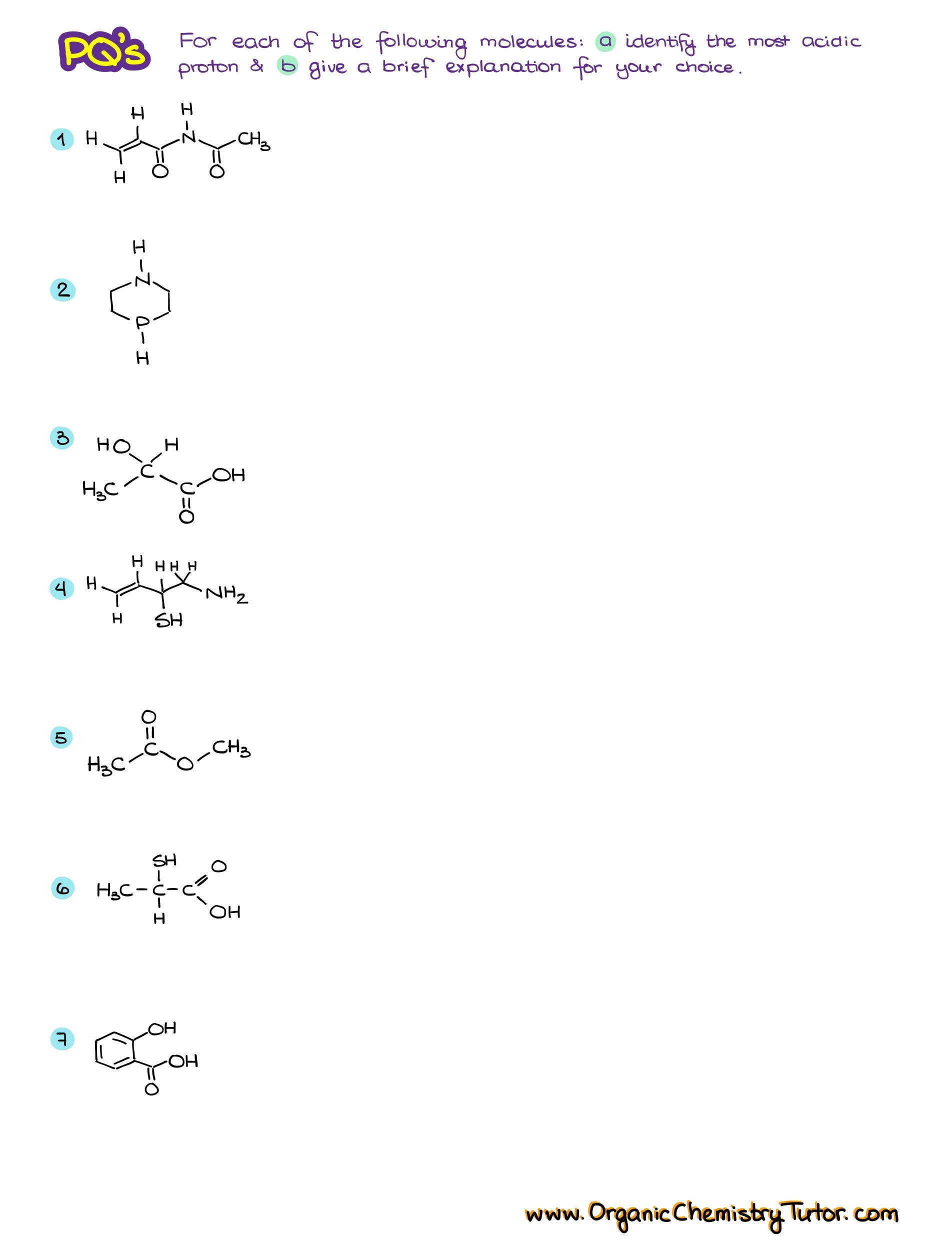
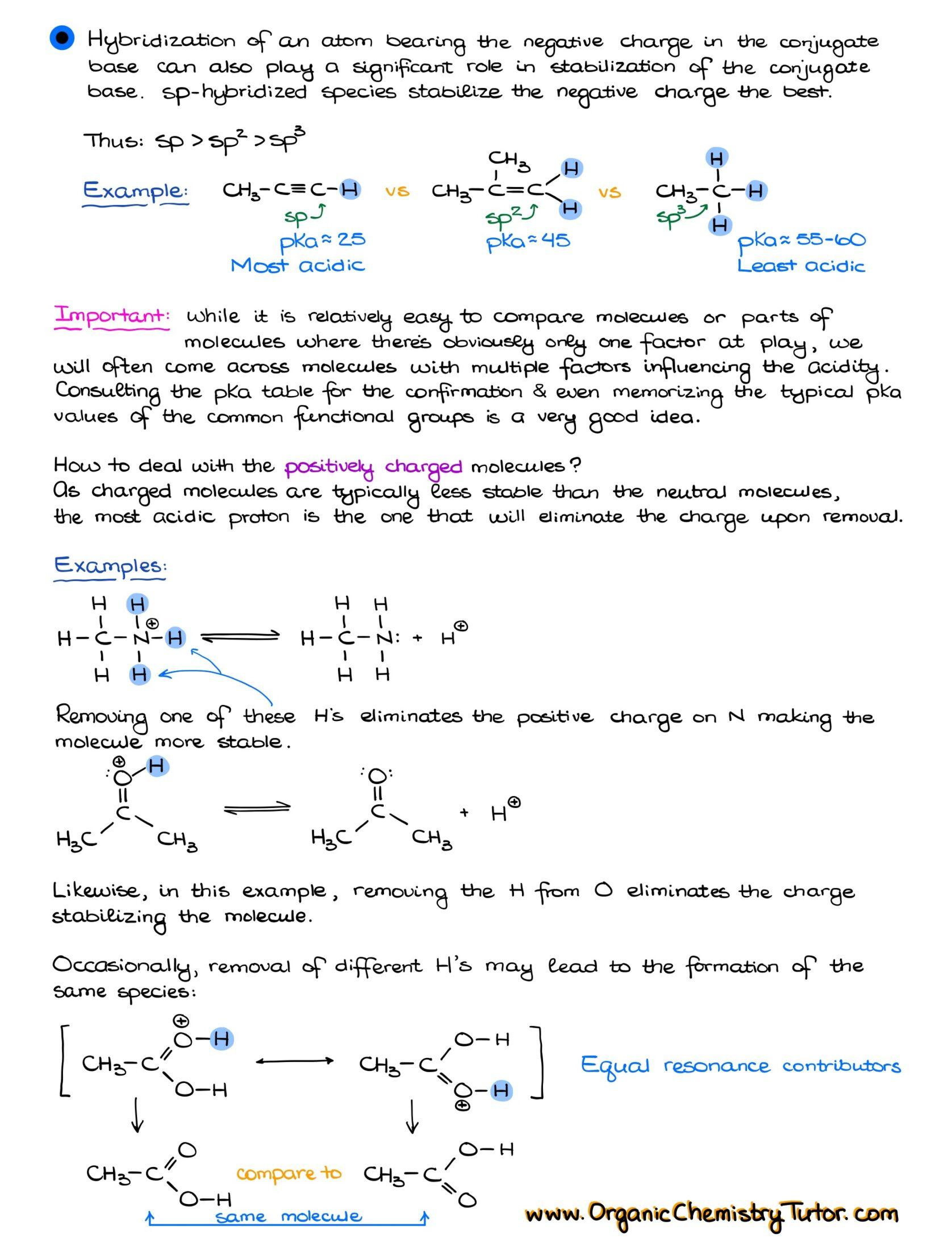
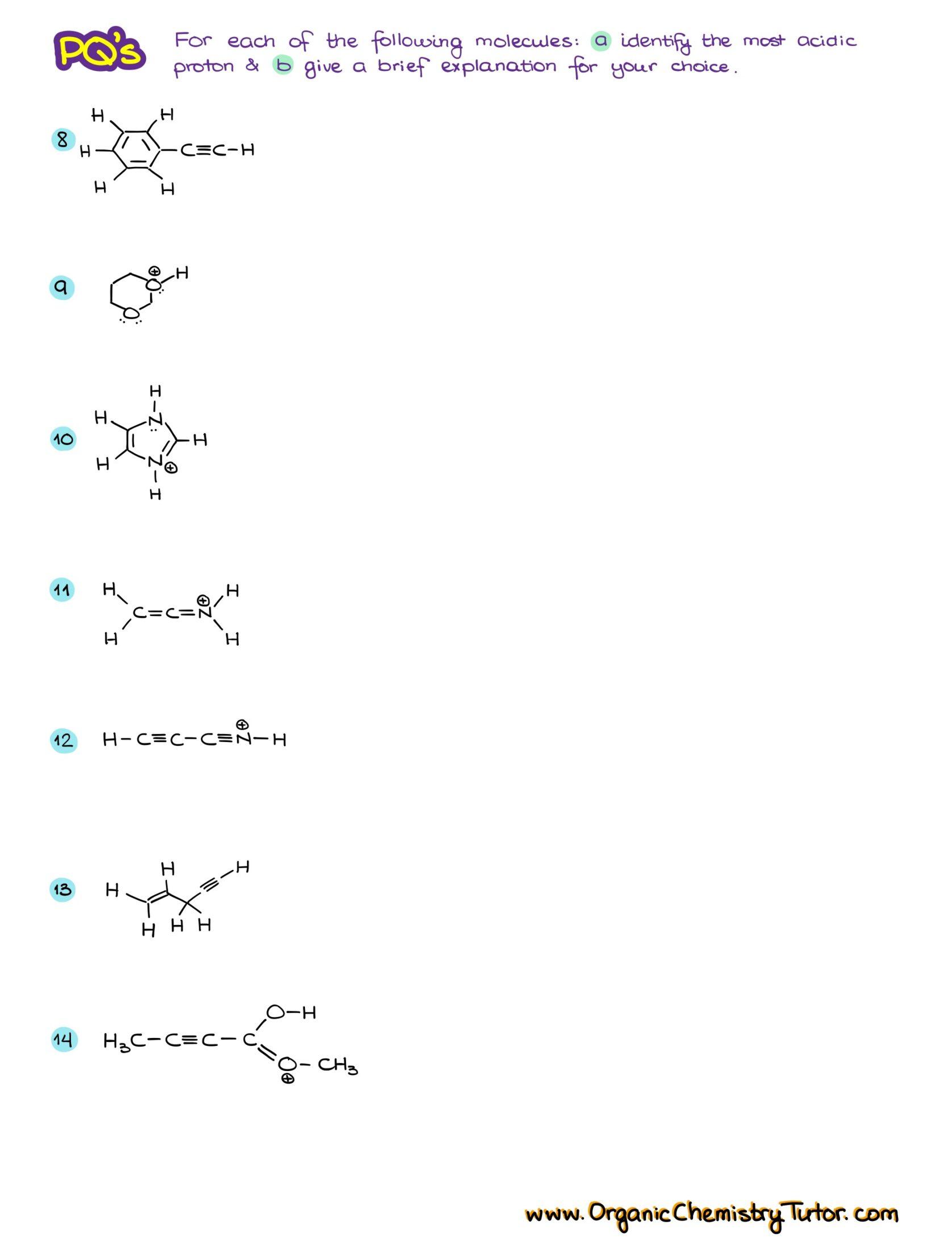
Source: https://t-tees.com
Category: WHICH