<?xml encoding=”utf-8″ ?>
To a creature that only lives for a few decades — less than a millionth of a percent of the Universe’s total age — a star is so long-lived that it may as well survive forever. Despite the fact that there are hundreds of billions of stars within our Milky Way alone, most of the humans that have ever lived have never seen a star die with their own unaided eyes. Here in our own Solar System, our parent star, the Sun, is already nearly 4.6 billion years old, yet it will be another 5-to-7 billion years before our Sun enters its final evolutionary stages: when it will become a red giant, expel its outer layers, and contract down to become a white dwarf.
You are viewing: Which Of These Stars Has The Longest Lifetime
But stars come in great varieties as far as their mass, color, and lifetimes go. Even though our Universe has seen an impressive 13.8 billion years pass since the start of the hot Big Bang, forming more than a sextillion (~1021) stars in the part that’s observable to us over that duration. Although many of those stars have already lived-and-died, most of the stars that have formed are still alive, and most living stars will outlive the Sun by a significant amount.
It’s enough to make one wonder: what’s the longest a star can live, and how long will our Universe continue to have stars? As of 2023, these are questions that science is up to the challenge of answering.
<?xml encoding=”utf-8″ ?>
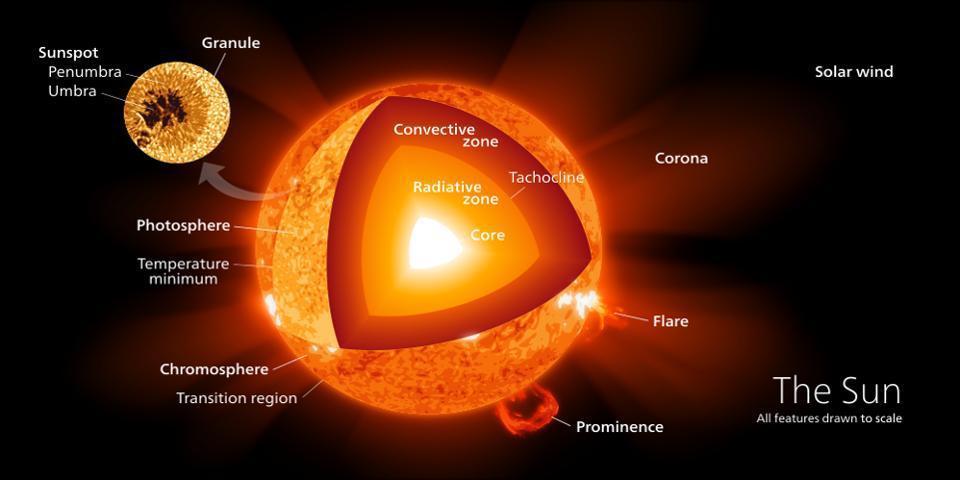
Inside of stars like our Sun, there are two incredibly strong forces that balance one another out all throughout its interior:
- the force of gravity, which works to pull every particle inside the star irresistibly toward the center,
- and the outward pressure from radiation, which arises due to the nuclear fusion reactions occurring in the star’s core.
If these forces, overall, are out of equilibrium, the star would either expand or contract in response until an equilibrium state is reached.
This helps us understand why, at the high end of the mass spectrum, the more massive a star is, the larger it tends to be. Stars undergo nuclear fusion in their cores: wherever the temperature exceeds 4 million K, which is the (approximate) temperature threshold to initiate hydrogen fusion in stars. However, higher temperatures lead to much greater rates of fusion. In the Sun, the center reaches up to 15 million K, and with these higher rates of fusion come greater temperatures and — as a result — a greater size for our star. If we look at how stellar mass, stellar temperature, and stellar size are related, we can see that as we increase a star’s mass, the temperature and size rise dramatically in response.
<?xml encoding=”utf-8″ ?>
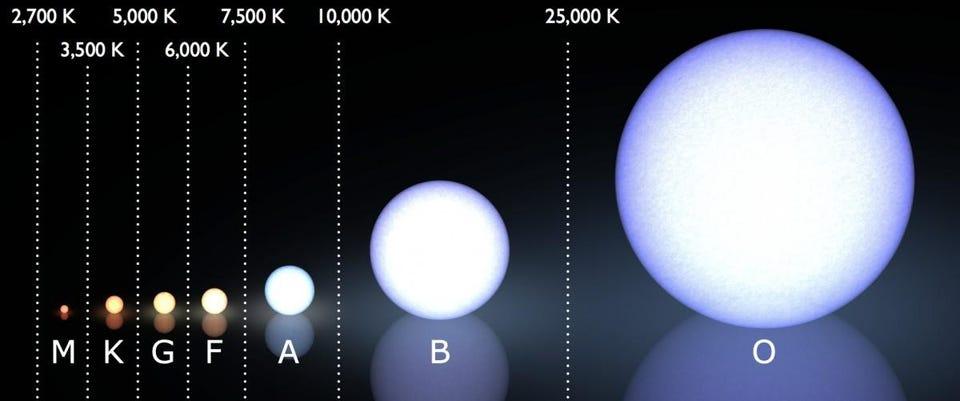
These relations, between a star’s mass and their color/temperature and size, are more severe than most people think. We often say, “The flame that burns twice as bright lives just half as long,” and that’s true: for flames that get their energy from equivalent stores of fuel. For stars, however, the situation is far more severe than for ordinary flames. If we were to compare three stars to one another:
- one that was the mass of the Sun,
- one that was twice the mass of the Sun,
- and one that was ten times the mass of the Sun,
we would find some tremendously different properties between them.
A Sun-like star has an estimated total lifetime of around 12 billion years, a size of 1 solar radius, a brightness of 1 solar luminosity, an average surface temperature of around 6000 K, and a whitish color to it.
A star that’s twice the mass of the Sun has an estimated lifetime of about 1.5 billion years, a size of ~1.7 solar radii, a brightness of about 25 solar luminosities, an average surface temperature of around 10,000 K, and a blue-white color to it.
Read more : Which Of The Following Is Typically True Of Weak Signals
And a star that’s 10 times the mass of the Sun has a total lifetime of only around 20-40 million years, a size that’s about 9 times the radius of the Sun, a brightness of around 25,000 solar luminosities, a surface temperature of 23,000 K, and a bluish color to it.
<?xml encoding=”utf-8″ ?>
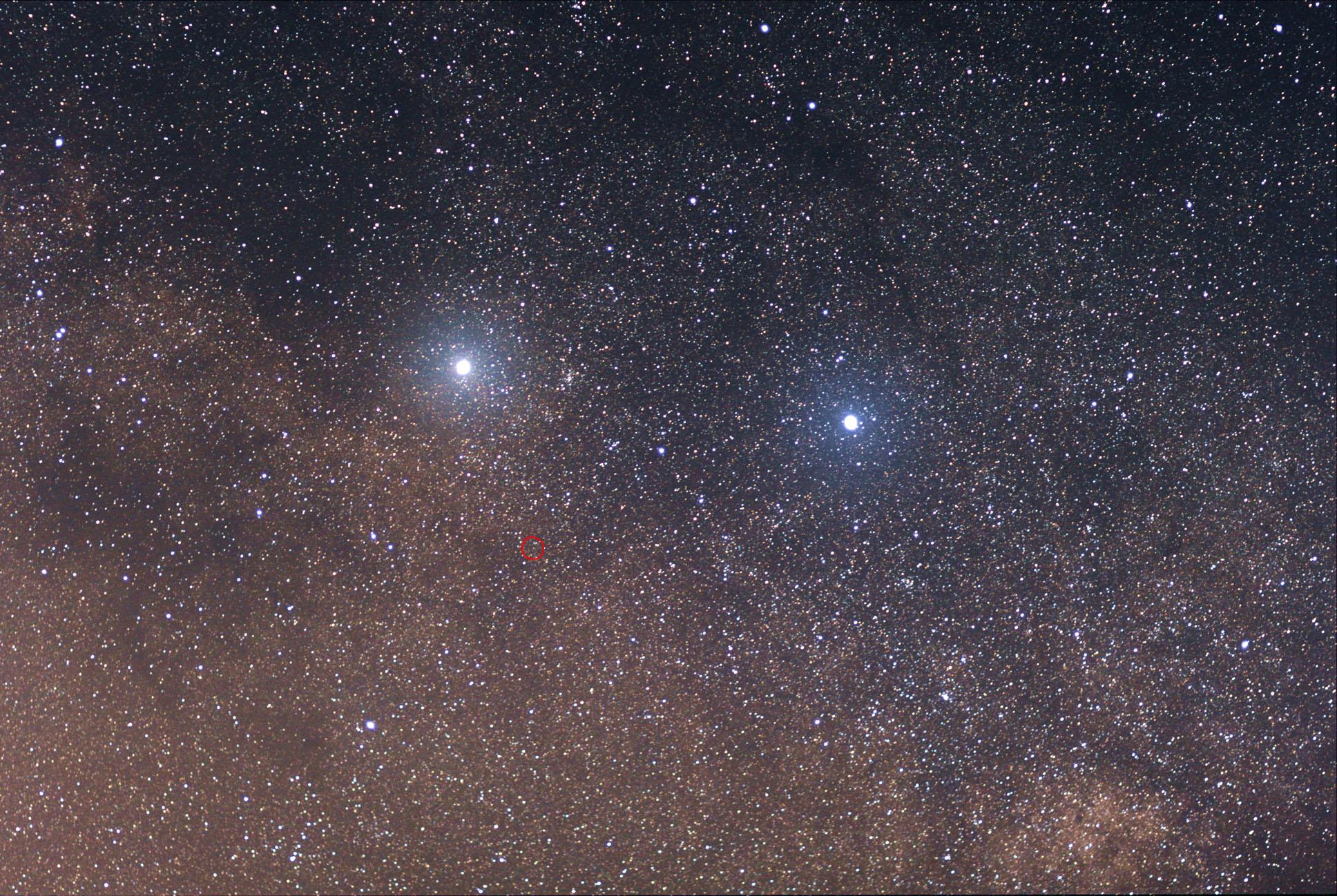
As a general rule of thumb, the lifetime of a star is inversely proportional to its mass cubed: a star twice as massive as the Sun lives just one-eighth as long, but a star only half as massive as the Sun should live for eight times the duration as the Sun. (The “cubed” is an approximation, as the relationship tapers off to the mass to the 2.5 power at the high-mass end, and grows to mass to the 4th power at the low-mass end.) In other words, the less massive your star, the longer it can live for, while the most massive stars, despite having more fuel, will burn through it much more quickly, and will have the shortest lifetimes of all.
It’s estimated that the highest mass stars that form, of hundreds of times the mass of the Sun (or perhaps even more), will endure for only 1-2 million years before dying in a catastrophic cataclysm, such as a supernova or hypernova explosion. As we go to lower and lower masses, however, we find that stars:
- burn through their fuel more slowly,
- live for longer amounts of time,
- die less violent deaths,
- and have more time to transport material from deep within the star to the outer layers, and vice versa.
Therefore, if we want to understand the longest-lived stars of all, we have to turn our attention to the least massive stars of all: the red dwarfs, which all have a different fate than our own Sun will.
<?xml encoding=”utf-8″ ?>

The Sun is our most well-studied star of all, and it turns out that somewhere between 20-25% of all stars are similar to it. If a star weighs in somewhere between 40% of the Sun’s mass all the way up to eight times as massive as the Sun, it’s going to have a very similar life cycle to our own star.
- It will fuse hydrogen into helium in its core during most of its lifetime,
- then, when its inner core runs out of hydrogen, the core begins to contract,
- which heats the star up, causes it to expand, and allows it to begin burning hydrogen in a spherical shell surrounding the inert core,
- and then the core undergoes what’s called the “helium flash,” where internal temperatures rise above a threshold of ~26 million K, enabling the start of helium fusion,
- and finally, when the inner core runs out of helium, the star blows off its outer layers to form a planetary nebula, while the remnant of the core contracts down to form a white dwarf.
Stars like the Sun, at the low-mass end of the spectrum, can have lifetimes that approach a whopping 200 billion years: more than 10 times the present age of the Universe.
But the technical definition of a star, to an astronomer, is “any object that undergoes hydrogen fusion in its core.” And the majority of stars, perhaps as many as 75-80% of all stars, fall into the red dwarf category: stars whose masses are below 40% the mass of the Sun, but who still fuse hydrogen into helium in their cores.
<?xml encoding=”utf-8″ ?>

These red dwarf stars, which can have as little mass as about 7.5-8% the mass of the Sun, look very different from the stars we’re used to in a number of important ways. Proxima Centauri, the nearest example of a red dwarf, has just 12% of the Sun’s mass.
- They’re relatively small: often barely larger than the planet Jupiter, which itself is less than 10% the radius of the Sun. Proxima Centauri has only 15% of the Sun’s radius.
- They’re faint and dim, outputting very little visible light compared to a Sun-like star. Proxima Centauri, for example, at 12% the mass of the Sun, puts out only 1-part-in-20,000 of the Sun’s visible light.
- They’re cooler and primarily radiate in the infrared, rather than in the visible portion of the spectrum. Proxima Centauri has a temperature of merely 3000 K, and radiates only 0.16% of the Sun’s total energy.
But what truly makes a red dwarf remarkable to many astronomers is the fact that it burns through its nuclear fuel so slowly, gradually, and gently, that these stars are what we call fully convective. Particles within a star don’t simply remain stationary, but can move around a little bit, as energetic particles from the interior can be transported to the outside, and cooler particles closer to the exterior can sink to the interior. This happens inside the Earth’s mantle; this happens in the atmospheres of giant planets; and it happens all throughout the interior of red dwarf stars.
<?xml encoding=”utf-8″ ?>

Read more : Which Way To Turn Distributor To Advance Timing
Whereas a Sun-like star has a large radiative zone in between the core and the outer convective zone, these common, low-mass stars are fully convective. That means that the time it takes particles to move in-and-out of the core is less than the time it takes for nuclear fusion to burn the core hydrogen fuel to completion. As a result, whereas a Sun-like star will fuse the hydrogen in its inner core to completion and then evolve to the next phase of its life, eventually expelling the unburned hydrogen in its outer layers, a red dwarf star will transport its nuclear material in-and-out of the core several times over its lifetime, eventually burning 100% of its internal hydrogen to completion.
Because of its lower mass relative to Sun-like stars and lower core temperature, red dwarfs will never achieve the necessary core temperatures, even when they’ve exhausted their hydrogen and begin to contract, to initiate helium fusion in their cores. Whereas today’s white dwarfs have all formed from Sun-like stars and are primarily composed of elements like carbon, oxygen, neon, and heavier elements than that, these red dwarfs will burn through all of their hydrogen and then fully contract down to become white dwarfs without either:
- becoming a giant,
- initiating “shell” fusion,
- igniting helium in their cores,
- or expelling their outer layers in a planetary nebula.
They will simply form a degenerate ball of helium comparable to the size of the Earth: a helium white dwarf.
<?xml encoding=”utf-8″ ?>
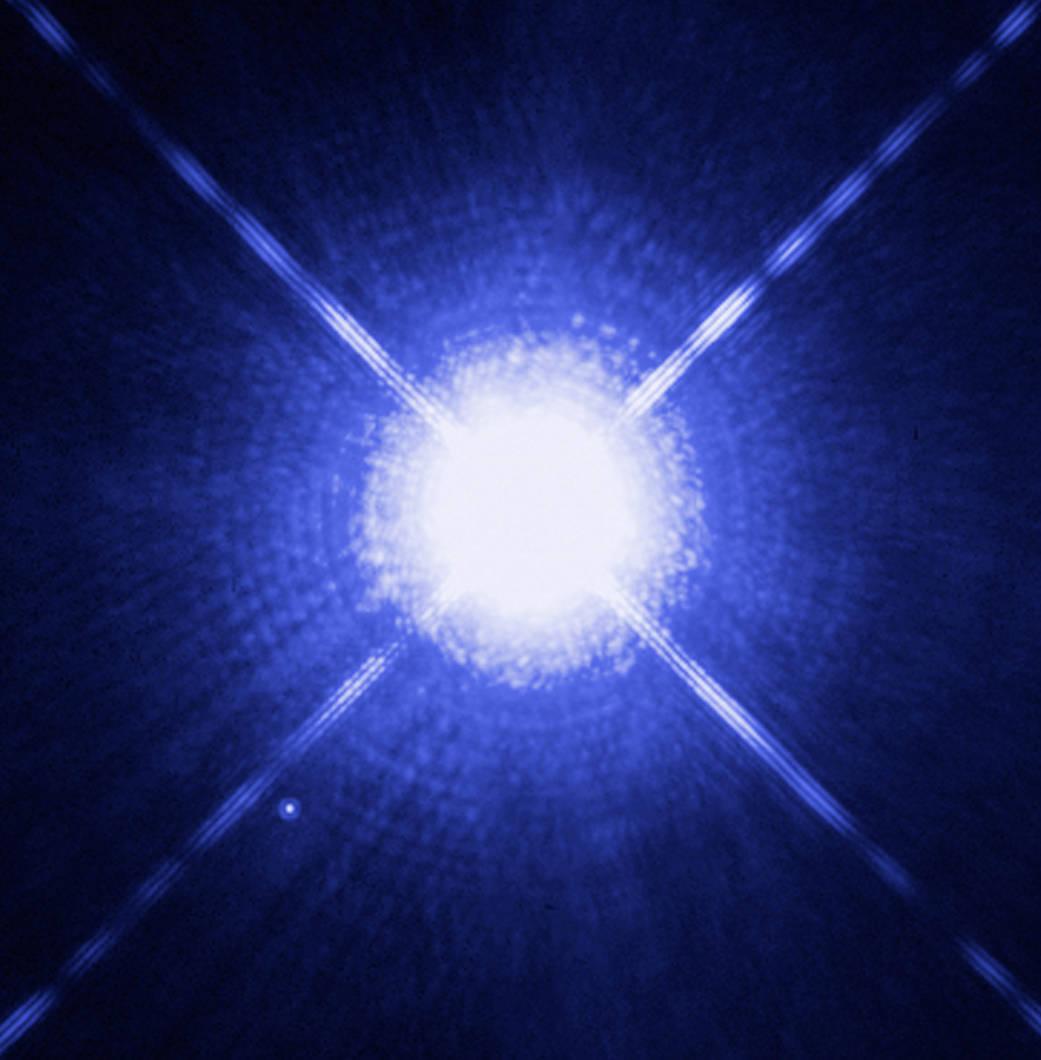
At the high-mass end of the red dwarf spectrum, these stars will live for a few hundred billion years before reaching their inevitable fates. However, it’s the lowest-mass stars that will live the longest. All the way down at the lowest-mass end of the stellar spectrum, where stars are only 7.5-8% the mass of the Sun (or about 80 Jupiter masses), these stars are no longer balanced by internal radiation counteracting the force of gravity; their sizes are primarily determined by the physics that governs atoms, the same as it would for a gas giant planet. In fact, the lowest-mass red dwarf star known, 2MASS J0523−1403, comes in at:
- 68 Jupiter masses (with an uncertainty of ±13),
- with a temperature of only 2000 K,
- emits just 0.014% of the Sun’s total luminosity,
- and is merely 1% larger in radius than the planet Jupiter.
It is so faint in visible light that it was only discovered by telescopes in the infrared, despite being relatively close by at only 41.6 light-years away. At the very low-mass end of being a true star, they may wind up being no larger than the largest gas giants found in our own Solar System.
<?xml encoding=”utf-8″ ?>
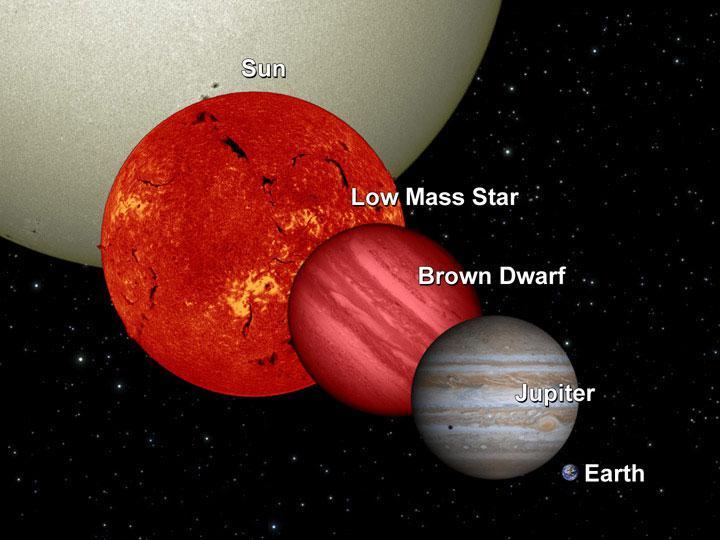
But how long will the lowest-possible mass red dwarf star live for? Assuming that nothing interferes with its life cycle, meaning:
- no other stars merge or interact with it,
- no companions siphon mass away from it,
- and nothing severely perturbs or disrupts it,
we’re talking about many trillions of years. There are severe uncertainties when it comes to estimating exactly how long such a star can live, but the minimum estimate is about 20 trillion years, with the maximum estimate rising up to around 380 trillion years. That’s a really, really long time!
But that doesn’t necessarily mean that, 380 trillion years from now, there will be no more stars visible in the night sky any longer. There are three reasons for this.
- Even though the star-formation rate, overall, has been decreasing for the past ~11 billion years of our cosmic history, new stars are continuing to form in gas-rich regions, which exist in our Milky Way and all throughout the Local Group.
- The Milky Way and Andromeda are headed for a great galactic merger, which will trigger an immense number of new stars some 4-7 billion years from now, and many of them will have incredibly low masses.
- But on even longer cosmic timescales, the Universe is filled with “failed stars” known as brown dwarfs, many of which exist in binary systems. When they inspiral and merge with one another, two brown dwarfs of sufficient mass can merge to produce a new red dwarf star, which can then burn for up to the maximum possible lifetime for a star.
<?xml encoding=”utf-8″ ?>
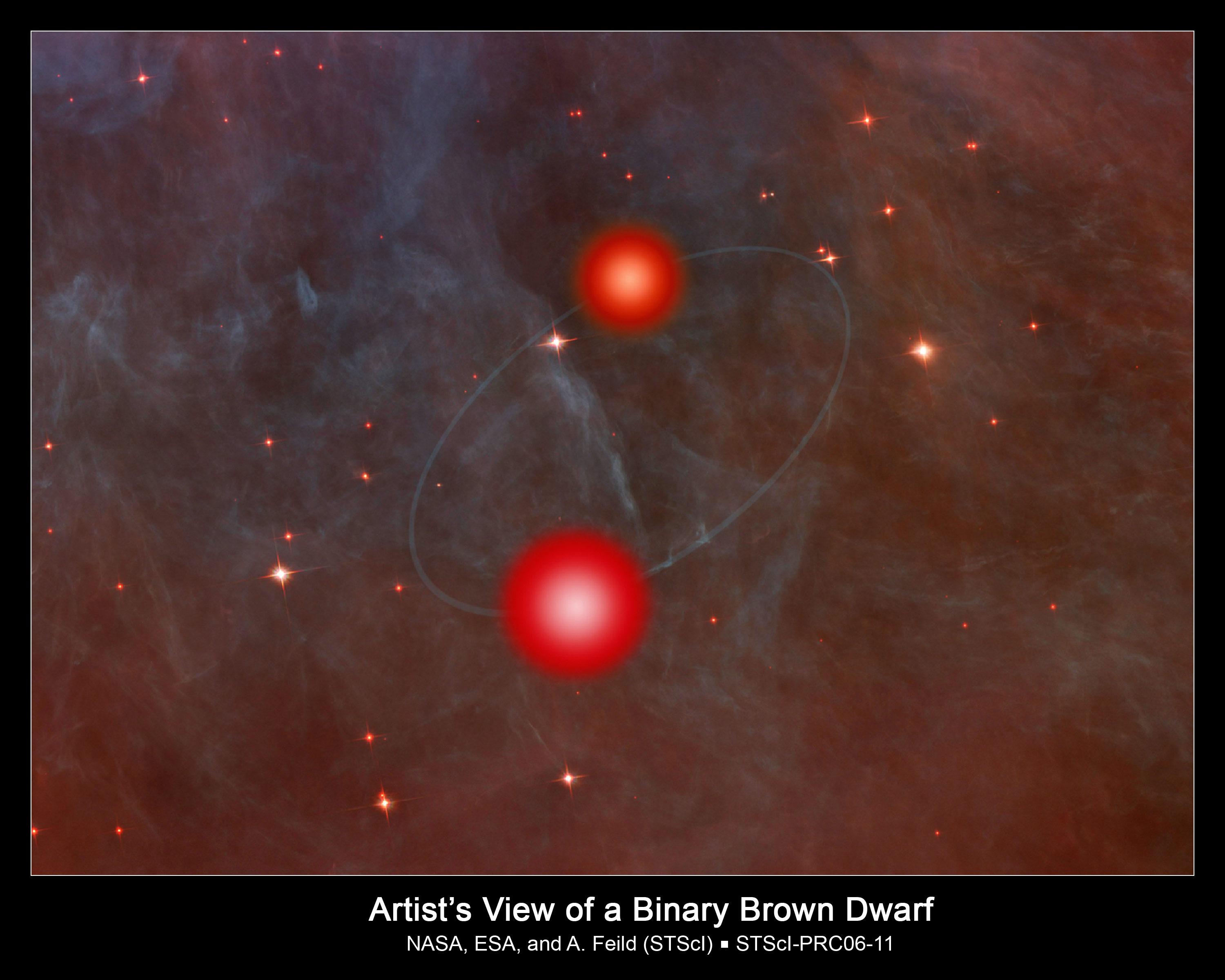
In other words, of the stars that exist right now, the longest-lived ones will survive for tens to hundreds of trillions of years, with a maximum possible lifetime of around 380 trillion years. But the Universe is still forming stars, and will likely still be forming stars in some capacity many trillions of years from now. Even once the galaxies of the Local Group have all merged together; even after the last vestiges of our cosmic gas is gone; even after dark energy has accelerated all the galactic groups and clusters beyond our own away, we will still have brown dwarf stars merging together.
When two brown dwarfs merge and their total mass crosses that ~80 Jupiter-mass threshold, a red dwarf will result, and a new star will come into existence. With a lifetime of trillions of years (up to a maximum of 380 trillion years), there will someday be a last, final star to form that’s visible to an observer in our Local Group. Although it’s hard to fathom such timescales, there may even be one or more stars that are shining several quintillion years from now: billions of times the present age of the Universe.
Although our Universe may be inevitably trending toward a heat death — a state of maximum entropy from which no further energy can be extracted — our Universe will continue to have stars for an incredibly long time to come. Nailing down exactly how long we can expect the longest ones to live is an area of research we’ve made a tremendous amount of progress on, but the ultimate answer still remains an unknown.
Source: https://t-tees.com
Category: WHICH